Quantum computing is a fast-growing field, but there is still a significant need in research and development to deliver on the promise of quantum advantage. The NISQ era (otherwise known as the Noisy Intermediate-Scale Quantum era) describes the field’s current state. With imperfect hardware, the stability of quantum processors and the coherence time of qubits (the length of time that information survives) is still relatively short-lived. Errors and decoherence decrease the system’s ability to perform useful computations. Thus, a key challenge is manipulating the quantum states of a growing number of qubits with precision and synchronicity on such short timescales.
The Quantum Systems Accelerator (QSA) has developed a technology roadmap of electronics controls. Electronic controls are the hardware and firmware that control and measure qubits through signals and pulses. By looking at the specifications of control systems that will be required across QSA’s computational platforms, the roadmapping exercise brought together leading experts from member institutions to establish common goals. It also served as an opportunity for researchers to share lessons learned about each platform and map out specialized solutions. QSA will share a follow-up article based on this roadmap which, once published, will help guide industry’s efforts in bringing advanced technologies to market.
Setting a shared direction
One challenge for QSA is developing three distinct quantum computational platforms with control systems that vary by qubit technology. For example, in superconducting systems, electronic controls turn baseband signals into microwave pulses to drive or interrogate the qubits. In another case, acoustic and optical modulators translate electric signals in trapped ions systems into a modulated optical signal directed towards the ions.
QSA Thrust Lead Mollie Schwartz is one of the researchers from MIT Lincoln Laboratory that led the electronics roadmap. With deep expertise in superconducting qubit systems, Schwartz pursues research on 3D integration and quantum algorithm implementation. Schwartz explained:
“We are diving deep into several different modalities of quantum computing at QSA. The value-added of this roadmap comes from having a broad perspective of all the technologies and knitting together the insights and the wealth of expertise that exist across all QSA institutions. This roadmap is a mechanism for a shared vision and highlighting areas where there can be closer collaboration across technology areas.”
This approach is a defining feature of QSA and its consortium of partnerships with other institutions and companies across the globe. Schwartz offered her insights about this need for a shared vision.
“In a classical computing setting, there have been a number of paradigmatic transitions in how a bit of information is physically stored, from punch cards to vacuum tubes to the transistors that eventually enabled the computing revolution. We don’t yet know which, if any, of these platforms is going to be the transistor of the quantum world, so we are pushing forward on all of them simultaneously.”
Identifying Commonalities
Dan Stick, QSA Thrust Lead hailing from Sandia National Laboratories, also led the roadmapping exercise. Stick is a recognized leader in micro-fabricated surface ion traps for quantum computing. He described how the QSA roadmap enabled researchers to understand and acknowledge the distinctive requirements for each hardware platform and how these differences impact quantum controls.
“There are enough differences among qubits systems that make it hard to imagine that a common piece of hardware will satisfy everybody. For example, you can’t just take one control system and apply it to all quantum technologies. But there are enough similarities that at least a subset of the hardware, firmware, and software can be shared across platforms. So we can leverage one person’s work for the QSA program and beyond that for the benefit of the broader community.”
QSA’s efforts to co-design electronic quantum controls are among the many integrated program-wide approaches to find the best path to quantum advantage. By focusing on what enables and what limits different quantum controls and what new high-fidelity technologies are necessary to develop, QSA catalyzes U.S. leadership in quantum information science. Unless robust controls are in place for each qubit technology, the quantum gate fidelities will not be sufficient for solving the complex science problems that quantum computers are ideally suited for. Stick said:
“Without these types of high-performance control systems, I don’t think we can reach that next level of performance for quantum computers that enables us to do higher fidelity and more accurate quantum algorithms, which then produce interesting science. So once you have more and more qubits, you have to get into this realm of electronic controls. It’s absolutely necessary to focus on this for future progress in quantum computing.”
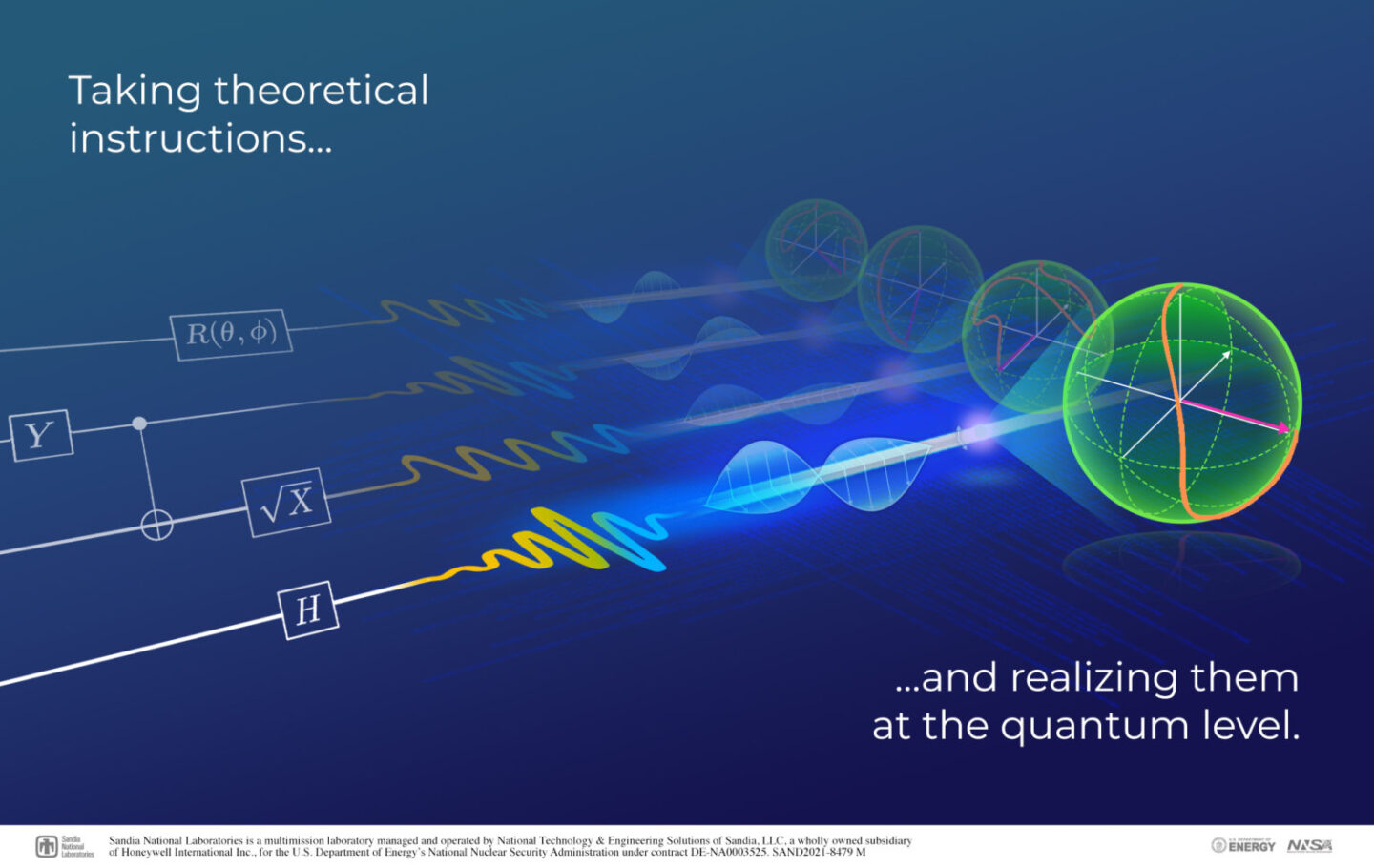
Driving Innovation
The research and development of electronic controls challenges researchers across the board, some of whom are still wrestling with the fundamental questions about materials science and custom fabrication. Sandia’s Dan Lobser participated in the roadmap as a QSA researcher focused on trapped ions.
Lobser explained that hardware devices such as the RFSoC (radio frequency system on chip) and FPGA (field programmable gate arrays) can modulate pulses and signals for superconducting and trapped-ion systems. These devices, however, were not originally made for quantum computing. They must be tailored for different control methodologies to satisfy the necessary performance requirements in frequency, time, stability, and bandwidth.
“For trapped ions, the challenge is not the fabrication of qubits because we have qubits which exist naturally and are pretty much ideal. It’s dealing with the world around them, which can shift their energy levels and degrade our gates. This noise presents us with one of the most important problems in making a scalable quantum computer. We need to be able to stabilize external systems in order to prevent errors or account for these errors by dynamically canceling them out.”
QSA researchers continue searching for methods to develop electronic controls that possess the flexibility to calibrate signals and pulses. In the case of Lobser’s efforts, he’s also exploring the integration of a device that incorporates a decision-making protocol and which is able to self-adjust based on a set of algorithms.
“We want to design hardware that gives as much flexibility as possible in generating these [signal] waveforms in a way that provides us with, essentially, as many options for gates as possible. Our approach is not to use standard commercial off-the-shelf components, such as arbitrary waveform generators, but to develop custom electronic designs that are specially tailored to these quantum platforms in order to handle some of the specific requirements that we at QSA and others in the community might need,” said Lobser.
In its mission to advance quantum information science, the QSA’s electronics control roadmap offers researchers the opportunity to share, learn, and innovate to pave the way towards practical and extensible quantum computers. To find out ways to collaborate or partner up with QSA, submit your inquiries at: https://quantumsystemsaccelerator.org/contact/
Founded in 1931 on the belief that the biggest scientific challenges are best addressed by teams, Lawrence Berkeley National Laboratory and its scientists have been recognized with 14 Nobel Prizes. Today, Berkeley Lab researchers develop sustainable energy and environmental solutions, create useful new materials, advance the frontiers of computing, and probe the mysteries of life, matter, and the universe. Scientists from around the world rely on the Lab’s facilities for their own discovery science. Berkeley Lab is a multiprogram national laboratory, managed by the University of California for the U.S. Department of Energy’s Office of Science.
DOE’s Office of Science is the single largest supporter of basic research in the physical sciences in the United States, and is working to address some of the most pressing challenges of our time. For more information, please visit energy.gov/science.
Sandia National Laboratories is a multimission laboratory operated by National Technology and Engineering Solutions of Sandia LLC, a wholly owned subsidiary of Honeywell International Inc., for the U.S. Department of Energy’s National Nuclear Security Administration. Sandia Labs has major research and development responsibilities in nuclear deterrence, global security, defense, energy technologies and economic competitiveness, with main facilities in Albuquerque, New Mexico, and Livermore, California.
The Quantum Systems Accelerator (QSA) is one of the five National Quantum Information Science Research Centers funded by the U.S. Department of Energy Office of Science. Led by Lawrence Berkeley National Laboratory (Berkeley Lab) and with Sandia National Laboratories as lead partner, QSA will catalyze national leadership in quantum information science to co-design the algorithms, quantum devices, and engineering solutions needed to deliver certified quantum advantage in scientific applications. QSA brings together dozens of scientists who are pioneers of many of today’s unique quantum engineering and fabrication capabilities. In addition to industry and academic partners across the world, 15 U.S. institutions are part of QSA: Lawrence Berkeley National Laboratory, Sandia National Laboratories, University of Colorado at Boulder, MIT Lincoln Laboratory, Caltech, Duke University, Harvard University, Massachusetts Institute of Technology, Tufts University, UC Berkeley, University of Maryland, University of New Mexico, University of Southern California, UT Austin, and Canada’s Université de Sherbrooke. For more information, please visit https://quantumsystemsaccelerator.org/