An active area of multidisciplinary exploration at the Quantum Systems Accelerator (QSA) is finding suitable materials to build increasingly complex quantum processors. Many of the current roadblocks in quantum information science and technology stem from disturbances and defects in the materials used to fabricate qubits across the leading platforms: neutral atoms, trapped ions, and superconducting circuits.
QSA scientists study sophisticated two-dimensional (2D) materials that could potentially increase the coherence time of qubits in superconducting circuits. Their research leverages broad expertise at different QSA partner institutions and U.S. Department of Energy (DOE) research facilities, including the Advanced Light Source and the Molecular Foundry at Lawrence Berkeley National Laboratory (Berkeley Lab).
LEVERAGING 2D VERSATILITY
Josephson junctions – the quantum equivalents of a transistor – are the building blocks of superconducting qubits. They result from a thinly layered sandwich of a non-superconducting material (insulator) between two layers of superconducting material, enabling the movement of an electrical charge through the material with little to no resistance. A vast majority of Josephson junctions typically use aluminum oxide as the insulator sandwiched between layers of aluminum. However, the traditional bulk materials – aluminum and niobium, used on classical silicon chips and Josephson Junctions – are potentially not the best materials for fabricating superconducting circuit processors. The material’s defects hinder the flow of electrical currents.
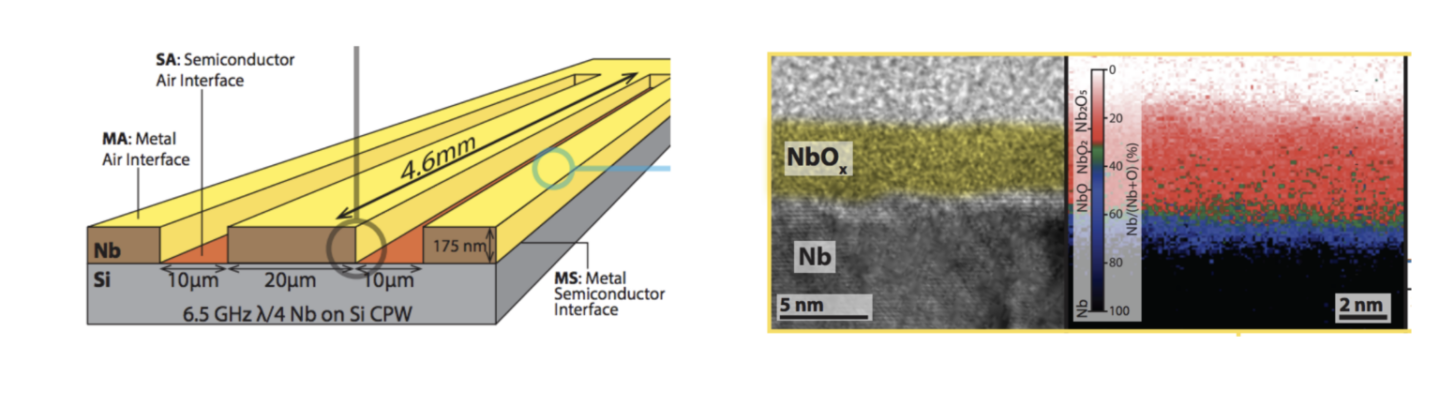
In contrast, 2D materials are extremely thin and versatile, with an average thickness of a few nanometers (nm) or less, and they tend to form in layers. Transition metal dichalcogenides (TMDs) hold great promise as a 2D material set for superconducting processors. TMDs can naturally be fabricated into very thin layers with a well-defined crystalline structure, so they can be stacked one on top of the other as a single crystal. This approach builds a well-defined nanostructure with potentially far fewer defects.
TMDs also have a series of important chemical and electronic properties that are often associated with reducing the impacts of defects. The dynamics are often subject to topological protection – a geometric property that increases the robustness of the quantum system itself.
Therefore, building Josephson Junctions with TMDs enables the fabrication of a superconducting quantum device without introducing new defects into the system.
QSA researcher James Analytis, a professor from the University of California, Berkeley and faculty scientist at Berkeley Lab, experiments with exotic materials that display quantum behavior. His studies include how electrons flow through 2D materials, particularly superconductors, magnets, and topological insulators (materials whose surface can conduct electric current but whose bulk restricts the movement of electrons).
Analytis gives the analogy of a well-defined wave traveling across a lake to illustrate the impact of material defects in quantum computing. The wave disperses as it hits rocks or objects along the way. Likewise, a slight breeze flowing over the lake might cause ripples and interactions on the lake’s surface. A similar disturbance occurs at an atomic and subatomic scale with the laws of quantum mechanics. The wave-like nature of electrons can be easily disturbed by a crystalline defect or by electromagnetic radiation, affecting them in unintended ways and causing decoherence – ripples in their quantum nature. In contrast, all of the atoms in TMD are in a perfectly regular array and the electrons behave in a coherent waveform.
“Not without its challenges, because TMDs have to be fabricated in highly controlled environments, and there is no production of TMDs at the industrial level, TMDs might have fewer defects than other materials. They’re just very clean and robust materials and there’s also inherently a multitude of options to build devices with these materials,” explained Analytis.
Some of the challenges in the R&D of 2D materials and their promise for Josephson junctions stem from the broad variety of chemistries and possible combinations. In this respect, QSA’s public mandate to mitigate risk and catalyze U.S. leadership as one of five DOE National Quantum Information Science Research Centers helps streamline research efforts.
“QSA has a very well-defined mission of the type of superconducting device it wants to create with a certain kind of functionality. So that focus really helps me in my work by identifying and prioritizing the important challenges and the real barriers to making the device. There are so many parameters in the development and study of new materials that this focus targets exactly what needs to be fabricated and how to get the device to work,” Analytis said.
DESIGNER MATERIALS, DESIGNER QUBITS
Berkeley Lab’s Sinéad Griffin, a QSA researcher and staff scientist at the Molecular Foundry, develops theoretical and computational methods to describe quantum materials. Aided by the advances in supercomputing capabilities, Griffin studies the variety of fundamental properties in 2D materials, including TMDs.
“We don’t really know yet what is the best material to use or the best combination of 2D materials because there are a lot of options available. There’s a lot of space to actively explore the fundamental questions that can impact what properties you want your qubit to have,” she said.
“In fact, although we’ve had 2D materials for a long time, getting them to the fabrication stage and to the high quality that we need for quantum computing applications requires us to both study what the fundamental properties of these 2D materials are and also start engineering them to be in the right form and the right properties. Many of the 2D materials’ electronic properties can change when stacking them one over the other. ”
Griffin explained how popular 2D materials, such as graphene, are not suitable for Josephson junctions on their own because they’re not inherently superconducting, even if stacked one over another. However, scientists recently found that when the angle between two graphene layers is twisted, the material becomes superconducting.
“It’s really exciting to think about what sort of designer properties we can get in these 2D materials where you’re not stuck (unlike with bulk materials) within a small region of space to engineer and enhance the functionality. We’ll be able to pick and choose the combination of materials and properties we want in our superconducting qubits,” said Griffin.
With advances in quantum information science, high-performance classical computing, and heterogeneous quantum-classical computing, QSA researchers such as Analytis and Griffin can study and experiment with broader materials systems and shorter feedback loops, translating to better materials for quantum hardware. Thus, QSA’s broad mandate to co-design the solutions for quantum advantage will increasingly require cutting-edge materials science and innovation.
Founded in 1931 on the belief that the biggest scientific challenges are best addressed by teams, Lawrence Berkeley National Laboratory and its scientists have been recognized with 14 Nobel Prizes. Today, Berkeley Lab researchers develop sustainable energy and environmental solutions, create useful new materials, advance the frontiers of computing, and probe the mysteries of life, matter, and the universe. Scientists from around the world rely on the Lab’s facilities for their own discovery science. Berkeley Lab is a multiprogram national laboratory, managed by the University of California for the U.S. Department of Energy’s Office of Science.
DOE’s Office of Science is the single largest supporter of basic research in the physical sciences in the United States, and is working to address some of the most pressing challenges of our time. For more information, please visit energy.gov/science.
Sandia National Laboratories is a multimission laboratory operated by National Technology and Engineering Solutions of Sandia LLC, a wholly owned subsidiary of Honeywell International Inc., for the U.S. Department of Energy’s National Nuclear Security Administration. Sandia Labs has major research and development responsibilities in nuclear deterrence, global security, defense, energy technologies and economic competitiveness, with main facilities in Albuquerque, New Mexico, and Livermore, California.
The Quantum Systems Accelerator (QSA) is one of the five National Quantum Information Science Research Centers funded by the U.S. Department of Energy Office of Science. Led by Lawrence Berkeley National Laboratory (Berkeley Lab) and with Sandia National Laboratories as lead partner, QSA will catalyze national leadership in quantum information science to co-design the algorithms, quantum devices, and engineering solutions needed to deliver certified quantum advantage in scientific applications. QSA brings together dozens of scientists who are pioneers of many of today’s unique quantum engineering and fabrication capabilities. In addition to industry and academic partners across the world, 15 U.S. institutions are part of QSA: Lawrence Berkeley National Laboratory, Sandia National Laboratories, University of Colorado at Boulder, MIT Lincoln Laboratory, Caltech, Duke University, Harvard University, Massachusetts Institute of Technology, Tufts University, UC Berkeley, University of Maryland, University of New Mexico, University of Southern California, UT Austin, and Canada’s Université de Sherbrooke. For more information, please visit https://www.quantumsystemsaccelerator.org/